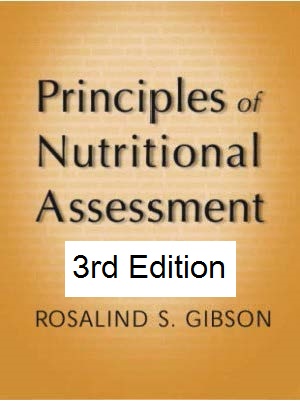
Nielsen, Forrest;
Principles of Nutritional
Assessment: Magnesium
3rd Edition, August 2024
Abstract
Magnesium is a cofactor for over 600 enzymatic reactions vital for life and is a controlling factor in nerve transmission, skeletal and smooth muscle contraction, cardiac excitability, vasomotor tone, blood pressure, and bone turnover. Thus, magnesium deficiency can have detrimental consequences that include impaired physical and mental well-being and risk for chronic disease. Before 1990, a nutritional magnesium deficiency was considered rare and to be present mainly in clinical conditions associated with gastrointestinal or renal loss of magnesium. However, numerous reports of an association between a low magnesium intake and chronic diseases, especially those associated with chronic inflammatory stress, has led to the recognition that mild or subclinical magnesium deficiency, also known as chronic latent magnesium (CLMD) deficiency, may be quite prevalent. As a result, magnesium has become a nutrient of public health concern and a simple, rapid, and reliable clinical measure is needed to assess for magnesium deficiency. Numerous methods of magnesium status assessment have been developed, but all have shortcomings that impact their use in the clinical setting to accurately assess magnesium status. The predominant method for assessing magnesium status at present is the determination of total serum or plasma magnesium. However, this method is not a sensitive indicator of body stores of magnesium. In the reference range of serum or plasma magnesium values, there is an interval in which individuals may have either CLMD or an adequate status. Combining measurement of total serum or plasma magnesium with both the determination of magnesium from dietary intake and urinary excretion appears to enhance the usefulness of this method to assess magnesium status. Recent models of ion-selective electrodes have enabled the measurement of plasma ionized magnesium, the physiologically active form, to be acceptably accurate and precise using whole blood. As a result, this method is becoming more prevalent in the clinical setting because the procedure is simpler and requires less blood than the measurement of total magnesium in plasma or serum magnesium. However, plasma ionized magnesium has shortcomings similar to those for total plasma and serum magnesium regarding the sensitivity of the reference range to determine CLMD accurately. Measurements of magnesium in erythrocytes, erythrocyte membranes, and mononuclear cells give a reasonable assessment of physiologically active body stores of magnesium. However, all these methods are laborious and prone to error, and hence only suitable for use in a research setting and not for routine clinical use. Urinary magnesium excretion is an excellent indicator of magnesium intake. However, a single urinary magnesium determination may not reflect magnesium stores because it responds so rapidly to changes in dietary intake. Hence, it is mainly appropriate for use in population studies, or in combination with other measures of status. The magnesium load test provides the most valid assessment of magnesium status. It has been used to identify magnesium deficiency in elderly individuals, in chronic alcoholism, and in several chronic diseases in which hypomagnesemia was not present. The test determines the percentage of urinary magnesium retained over a given period of time after parenteral administration of a magnesium load and yields an abbreviated balance determination. The test is invasive, time-consuming, and cumbersome; it requires close supervision for at least 24 hours after a magnesium load. Because of these drawbacks, it has been used mostly as a research tool. Several other methods used to assess magnesium status also have drawbacks that preclude their use as routine clinical methods. Muscle magnesium determination is extremely invasive and requires skill to obtain suitable samples for analysis. Buccal cell magnesium determinations also require skill in obtaining cells, and special expensive equipment for analysis. Magnesium balance requires controlled and consistent dietary intakes and careful collection of urine and stool over a lengthy study period. Fractional excretion of magnesium requires the determination of three variables and has no apparent advantage over other well-established methods. The magnesium depletion score needs further evaluation and validation, although seems to be most useful for establishing status in individuals with a chronic disease. However, all these methods do not have an established validated reference range that indicates magnesium adequacy or deficiency. At present, there is no simple, rapid, and reliable single clinical method to determine the presence of chronic, latent magnesium deficiency (CLMD). Determination of serum total or ionized magnesium remains the most acceptable choice in the clinical setting. The reliability of these measures of status could be improved if their values for the reference ranges indicative of deficient or adequate magnesium status are confirmed by other measures such as the determination of magnesium in both dietary intakes and urinary excretion. CITE AS: Nielsen, Forrest; Principles of Nutritional Assessment: Magnesium. https://nutritionalassessment.org/ magnesium/Email: frostynielsen@yahoo.com
Licensed under CC-BY-4.0
( PDF )
23c.1 Introduction
The human body contains about 760mg of magnesium at birth, 5g at age 4‑5 months, and 25g when adult (World Health Organization and Food and Agriculture Organization of the United Nations, 2004). There are three body pools of magnesium (Rude & Shils, 2006). One pool has a turnover rate of less than 28hours. This pool of extracellular magnesium, which includes blood, contains 0.8‑1.0% of body magnesium. The second pool has a turnover rate of about 11days. This pool is primarily intracellular magnesium, of which about 40% of the magnesium is found in soft tissues where it performs most of its essential functions. Skeletal magnesium is the third pool with a slow turnover rate measured in months or years in normal adults. Skeletal magnesium contains about 60% of body magnesium of which two-thirds is found within the hydration shell (bone mineral or poorly crystallized hydroxyapatite) and one-third on the crystal surface of the skeleton (Rude & Shils, 2006). Surface magnesium is readily exchangeable with serum and serves as a reservoir for the maintenance of magnesium for essential functions when intakes of magnesium are deficient.23c.2 Functions of magnesium
Divalent magnesium (Mg2+) is the fourth most abundant cation in the body and is second to potassium as the most abundant intracellular cation. Magnesium is a cofactor for over 600 enzymatic reactions vital to pathways including DNA, RNA, protein and adenosine 5'-triphosphate (ATP) synthesis; cellular energy production and storage; glycolysis; and cellular second messenger systems. The predominant enzymatic role of magnesium in these pathways involves an enzyme interacting with MgATP to complete a reaction. Magnesium also binds directly to enzymes to cause conformational change (allosteric activation) that gives a catalysis center for reaction to occur. Stabilization of ribonucleotides and deoxyribonucleotides by magnesium allow for DNA duplication, transcription, and maintenance, and transfer RNA function (Rude & Shils, 2006). Magnesium reacts with phosphates and carboxylates to stabilize membranes, affecting their fluidity and permeability which influences cellular ion channels, transporters, and signaling (Rude & Shils, 2006). Through regulating Ca2+ and K+ movement in and out of the cell, magnesium is a controlling factor in nerve transmission, skeletal and smooth muscle contraction, cardiac excitability, vasomotor tone, blood pressure, and bone turnover.23c.3 Absorption and metabolism
The jejunum and ileum are the primary sites of magnesium absorption; lesser amounts are absorbed by the cecum and colon. When dietary intakes of magnesium are near requirement, 45‑55% is absorbed. With intakes less than 50% of requirement, the percentage of magnesium absorbed may increase to 65‑70%. As dietary intake increases over requirement the percentage of magnesium absorbed decreases. For example, with an intake of 972mg, fractional absorption of magnesium was only 11% (Fine et al., 1991). Magnesium is absorbed via both active transport (transcellular) and passive diffusion (paracellular). Active transport occurs in the ileum, although apparently also in the cecum and colon through the action of magnesium transporters: transient receptor potential melastatin (TRPM) type 6 (TRPM6) and TRPM7 (de Baaij et al., 2015). Passive paracellular diffusion accounts for about 90% magnesium absorption and is dependent on a high concentration in the jejunum and ileum and a transcellular potential difference generated by sodium transport (Romani, 2013). Magnesium absorption can also be affected by other dietary components, including calcium, phosphorus, fiber, phytate, protein, and oxalates, all of which can decrease absorption, whereas vitamin D and its active metabolites, carbohydrates fermentable by bacteria, and oligosaccharides increase absorption (Nielsen, 2017). The primary organ regulating magnesium homeostasis is the kidney where about 10% of total body magnesium is filtered daily through the glomeruli with the nephron recovering 95‑99% of filtered magnesium. Passive diffusion associated with calcium, sodium, and water transport results in the reabsorption of about 10‑25% of the magnesium (de Baaij et al., 2015). Passive diffusion in the thick ascending limb of the loop of Henle accounts for about 50-70% of the magnesium reabsorption. The remaining 5‑10% of filtered magnesium is reabsorbed in the distal convoluted tubule via an active transcellular mechanism. The active transport channel in the kidney apparently involves TRPM6. When magnesium intakes are deficient, excretion of magnesium by the kidney decreases to 12‑24mg/day to conserve body magnesium. Diets high in sodium, calcium and protein, and caffeine and alcohol consumption all enhance magnesium excretion (Costello & Rosanoff, 2020).23c.4 Magnesium deficiency in humans
Signs and symptoms of severe magnesium deficiency include positive Trousseau's and Chvostek's signs; muscle spasms, fasciculations, and tremor; and personality changes such as apathy, depression, nervousness, delirium, and hallucinations (Rude, 1998). However, severe magnesium deficiency involving these signs and symptoms is rare and usually associated with predisposing and complicating disease states that cause low magnesium intakes or impaired intestinal or renal absorption. In conditions of long term moderate or mildly deficient intakes of magnesium or excessive excretion, magnesium is slowly released from bone to assure that vital essential functions are fulfilled. Nevertheless, there may still be a lack of magnesium required for some functions, but the signs associated with severe magnesium deficiency outlined above do not occur. This lack has been called chronic latent magnesium deficiency (CLMD) (Elin, 2010). Chronic latent magnesium deficiency is considered a factor contributing to several chronic diseases, especially cardiovascular disease, including ischemic heart disease, sudden cardiac death, heart failure, atrial fibrillation, and ischemic stroke (Costello & Rosanoff, 2020). The associated risk of CLMD with cardiovascular disease is through impaired glucose metabolism and insulin action, dyslipidemia, hypertension, chronic inflammation, and impaired vasomotor tone (Costello & Rosanoff, 2020). Chronic latent magnesium deficiency also may result in an increased risk for bone fractures and osteoporosis (Costello & Rosanoff, 2020). Apatite crystals formed in magnesium-deficient bone are increased in size, which makes bone more brittle, increasing the fracture risk. Magnesium deficiency can also reduce parathyroid hormone and 1,25-dihydroxycholecalciferol levels, which affect the regulation of magnesium-dependent calcium channels and changes in the release of inflammatory cytokines. Such effects enhance osteoclastic action and inhibit osteoblastic action, resulting in bone loss and an increased risk for osteoporosis. Other chronic diseases with which CLMD has been associated include osteoarthritis, chondrocalcinosis, depression, and migraine headaches (Costello & Rosanoff, 2020). In addition, CLMD has been found to cause chronic inflammatory & oxidative stress, indicating that through these effects, CLMD may contribute to chronic diseases, other than those noted above, in which inflammatory or oxidative stress facilitates their occurrence.23c.5 Food sources and dietary Intakes
Foods of plant origin provide about 50% of the magnesium intake for adults on mixed diets (Hunt & Meacham, 2001). Meats supply about 14‑16% and dairy products (including milk) supply about 34% of the magnesium intakes in U.S. adults (Hunt & Meacham, 2001). Rich food sources of magnesium include whole grains / cereal, nuts, pulses, green leafy vegetables, and dark chocolate. Intermediate sources of magnesium include cheese, meats, and most seafoods. Refined grains are poor sources of magnesium.23c.6 Effects of high magnesium intakes
Because the kidneys are very effective in the excretion of excessive magnesium, severe magnesium toxicity rarely occurs. When severe toxicity resulting in high blood magnesium occurs, the condition arises almost exclusively in individuals with kidney dysfunction or failure who are given magnesium salts or drugs such as laxatives or antacids. Symptoms of toxicity include lethargy, confusion, nausea, diarrhea, impaired breathing, hypotension, muscle weakness, and heart arrhythmias (Romani, 2013). Signs of hypermagnesemia also include low blood calcium and high blood potassium. The heart rhythm changes in magnesium toxicity are caused by abnormal electrical conduction which occurs at the nervous, muscular, and cardiac level. Severe magnesium toxicity caused by excessive intake through food has not been reported. High intakes of supplemental magnesium available in forms such as aspartate, citrate, chloride, gluconate, lactate, and oxide can cause adverse effects such as diarrhea, abdominal cramping, and nausea. A tolerable upper intake level (UL), defined as the highest level of daily intake that is likely to pose no risks of adverse health effects to almost all individuals in the general population, was set at 350mg of supplemental magnesium in the United States and Canada for children older than 8 years and adults by the (Institute of Medicine. 1997),23c.7 Occurrence of dietary deficiency
In the official Dietary Reference Intakes (DRIs) established for the United States and Canada (Institute of Medicine. 1997), the Estimated Average Requirements, (EAR, defined as average daily intake estimated to meet the daily requirements of 50% of healthy individuals) were set for individuals from aged 19 to 70 years and over at 330 to 350mg/day for males and at 255 to 265mg/day for females. These EAR values for adults are consistent with those set for adults in several other countries, as shown in Table 23c.1.US/Canada
Institute of Medicine (1997). | Age(y) | EAR | RDA |
Males | 19-30 | 330 | 400 |
31-70+ | 350 | 420 | |
Females | 19-30 | 255 | 310 |
31-70+ | 265 | 320 | |
United Kingdom Dietary Reference Values (1991). | Age | EAR | RNI |
Males | 19-75+ | 250 | 300 |
Females | 19-75+ | 200 | 270 |
Australia/New Zealand NZ Ministry of Health (2006). | Age | EAR | RDI |
Males | 19-30 | 330 | 400 |
31-75+ | 350 | 420 | |
Females | 19-30 | 255 | 310 |
31-75+ | 265 | 320 | |
WHO (2004). | Age | RNI | |
Males | 19-65 | NA | 260 |
65+ | NA | 224 | |
Females | 19-65 | NA | 220 |
65+ | NA | 190 |
23c.8 Indices of magnesium status
Because magnesium has so many critical functions, the body has strong mechanisms, such as reducing urinary excretion and releasing skeletal stores of magnesium, to assure that magnesium is readily available to fulfill those functions. In addition, mild or moderate magnesium deficiency is mostly asymptomatic. As a result of these characteristics, success in finding a cost-effective, time-efficient, and reliable indicator of magnesium status is lacking. Among the status indicators that have been evaluated are serum or plasma total magnesium, serum ionized magnesium, erythrocyte magnesium, erythrocyte membrane magnesium, blood mononuclear cell magnesium, urinary magnesium excretion, magnesium load test, sublingual magnesium, muscle magnesium, and fractional excretion of magnesium. An additional factor that may be useful in evaluating exposure to magnesium is dietary intake.23c.8.1 Serum/plasma magnesium
At present, total serum magnesium is the predominant clinical test to assess magnesium status, although plasma total magnesium is sometimes measured instead of serum magnesium. However, serum is preferred because anti-coagulants might be contaminated with magnesium or affect assessment methods. Serum magnesium determination is inexpensive and easily performed using atomic absorption spectrophotometry or colorimetric methods. The normal range for serum magnesium is 0.75‑0.95mmol/L. (Figure 23c.1). Hence, theoretically, serum concentrations above 0.75mmol/L should be considered magnesium sufficient, and those below 0.75mmol/L should be considered magnesium deficient, although, as indicated below, perhaps not rightly so (Costello et al., 2016). Serum magnesium concentrations are reportedly acceptable for determining a severe lack of magnesium intake or an elevated urinary excretion of magnesium resulting in a depletion of body stores. This finding is based on the results of a study in which exposure to extreme dietary magnesium deprivation (less than 12mg/day) resulted in a decline in serum magnesium from 0.80mmol/L to 0.61mmol/L in three weeks (Fatemi et al., 1991). However, in mild or moderate dietary magnesium deprivation, serum magnesium concentrations can remain in the normal range after several weeks, based on controlled metabolic unit studies of postmenopausal women whose dietary intakes were changed from adequacy (250mg/day) to moderate deficiency (80‑160mg/day) (Nielsen & Johnson, 2017). Nevertheless, the magnesium-deprived women showed signs indicative of magnesium deficiency that responded to magnesium supplementation, indicating their body tissues were becoming magnesium deficient. Results of these metabolic unit studies are consistent with clinical studies of patients with diabetes mellitus, alcoholism, and malabsorption syndromes, all of whom had serum magnesium concentrations in the sufficient range, yet had low magnesium concentrations in erythrocytes, mononuclear cells, and muscle (Ryzen et al., 1986; Nadler et al., 1992; Abbott et al., 1994; Rude & Olerich, 1996). These findings indicate that individuals with serum/plasma magnesium concentrations within the current reference range might be deficient enough to have a bearing on the risk for chronic disease.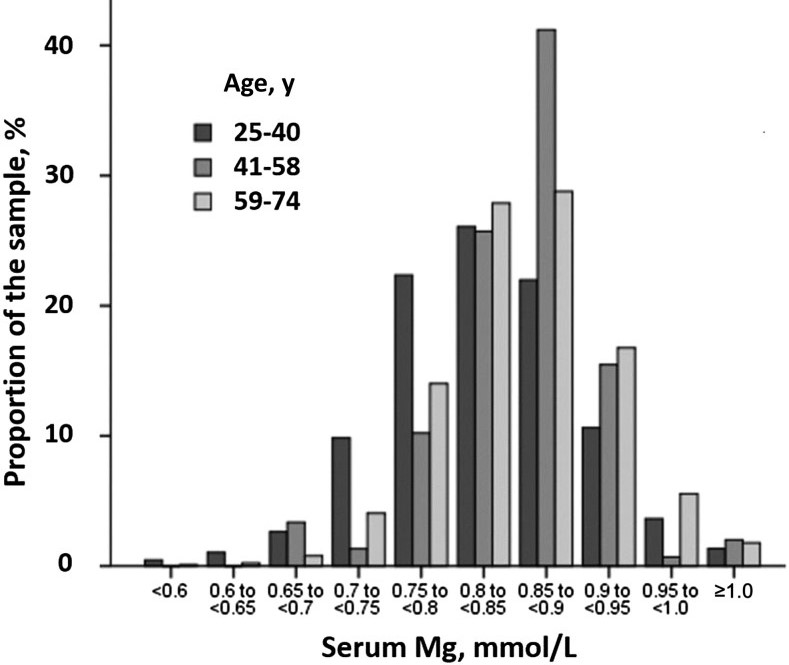
23c.8.2 Serum, plasma, and blood ionized magnesium
Ionized magnesium is the physiologically active form of magnesium that is transported across cell membranes and participates in metabolic interactions and enzymatic reactions. Thus, it has received attention as a better measure than serum total magnesium for indicating the status of magnesium for essential cellular functions in the body. Ionized magnesium is determined in serum, plasma, or whole blood samples by using equipment with ion-selective electrodes. The first ion-selective electrodes were challenging to use, cost-intensive, and had poor ability to discriminate ionized magnesium from ionized calcium; this inhibited the use of ionized magnesium as a status-assessment measure. However, recent models of ion-selective electrodes are cost-effective and have been shown to be acceptably accurate and precise in measuring only ionized magnesium. Originally, serum ionized magnesium was often determined, but at present, it is plasma magnesium that is mostly measured in whole blood; the ionized magnesium in erythrocytes is not measured. An advantage of using whole blood is that less blood and fewer procedures are needed for analyses. High pH and high concentrations of ionized calcium, lipophilic cations, and thiocyanate (caused by smoking tobacco) can interfere with the determination of plasma ionized magnesium by ion-selective electrodes (Ansu Baidoo et al., 2023). Plasma ionized magnesium as a status assessment measure has the same problems as those outlined for plasma total magnesium; the body also attempts to maintain magnesium concentrations in plasma at a level needed for vital functions through reduced urinary excretion and bone resorption. Factors that affect plasma total magnesium, such as diuretics, proton pump inhibitors, and various disease states, also affect plasma ionized magnesium. An additional issue is that a reference range for plasma ionized magnesium indicative of adequate magnesium status has not been firmly established and appears to vary depending upon the instrument used for determining a range. A systematic review and meta-analysis involving all types of instruments used found a 95% confidence interval for ionized magnesium in healthy adults to be 0.40 to 0.68mmol/L (Ansu Baidoo et al., 2023). Using this as a reference range indicates plasma ionized magnesium concentrations below 0.40mmol/L are most likely indicative of magnesium deficiency and those above 0.68mmol/L are hypermagnesemic. However, the meta-analysis also found that in patients with chronic diseases such as cardiovascular disease, type 2 diabetes, and hypertension associated with magnesium deficiency, the 95% confidence intervals for plasma ionized magnesium concentrations included those found for healthy individuals. This indicates, as noted for plasma total magnesium, that individuals in the designated adequate range for plasma ionized magnesium may also be magnesium deficient. Thus, additional measures of a dietary magnesium intake <250mg/day and a urinary magnesium excretion <80mg/day might help in identifying individuals who are very magnesium deficient even though they have plasma ionized magnesium concentrations in the low healthy reference range.23c.8.3 Erythrocyte magnesium
Magnesium exists in erythrocytes in free, complex, and protein-bound forms. Magnesium concentrations in erythrocytes are about three times greater than those in plasma and reflect chronic rather than acute magnesium status because of the long lifespan of erythrocytes (120 days). Cellular magnesium is considered more reflective of magnesium status than circulating magnesium. However, erythrocyte magnesium concentrations have been reported as not correlating well with other tissue pools such as mononuclear blood cells or muscle (Ryzen et al., 1986; Elin, 1987). Thus, it is uncertain how well erythrocyte magnesium concentrations reflect body magnesium stores. Determination of cellular magnesium concentrations takes special procedures including centrifugation to separate blood into an erythrocyte bottom layer, an intermediate buffy-coat layer of white blood cells, and a top layer of plasma. Erythrocytes are then acid digested and the magnesium content determined by atomic absorption spectrometry. Care must be taken to avoid hemolyzing the blood sample when obtaining the erythrocytes as it would result in loss of magnesium to the plasma portion. A reference range for the concentration of magnesium in erythrocytes of 1.65 to 2.65mmol/L has been reported (Costello & Nielsen, 2017). In depletion-repletion metabolic unit studies, a change in magnesium status determined by expressing erythrocyte magnesium as mmol/mg erythrocyte membrane protein appeared to be better than when expressed per erythrocyte cells, packed cells, or hemoglobin (Nielsen et al., 2003, 2007a, 2007b). The range in values found in these studies for erythrocyte membrane magnesium was 2.26 to 3.25 nmol/mg protein. However, this method of measuring status requires additional steps involving hemolyzing collected erythrocytes followed by washing and collecting the erythrocyte membranes after isolating them from the erythrocytes (Dodge et al., 1963). The studies of Dodge and co-workers (Dodge et al., 1963) also determined erythrocyte ionized magnesium by hemolyzing the erythrocytes to release ionized magnesium for measurement by an ion selective electrode. However, determining the erythrocyte ionized magnesium content or expressing magnesium per cell or g hemoglobin did not appear to be any better for assessing cellular magnesium status than determining erythrocyte magnesium mmol/L packed cells. Further studies might find measuring red blood cell membrane magnesium useful in the research setting. Even though the assessment of body magnesium stores or CLMD may be slightly better using erythrocyte magnesium, the method of measurement is laborious and prone to error, that coupled with lack of a well validated reference range, preclude measurements of erythrocyte magnesium from being a useful clinical method for determining magnesium status. Erythrocyte membrane or packed cell magnesium determination may be useful in determining changes in magnesium status in long-term research studies of magnesium depletion and repletion.23c.8.4 Magnesium in mononuclear and other cell types
Experimental findings from rats showing that changes in magnesium concentrations in mononuclear cells (lymphocytes and monocytes) reflected those in cardiac and skeletal muscle (Ryan & Ryan, 1979) was a stimulus to examine whether these cells could be used for magnesium status assessment in humans. Between 1980‑2000, magnesium measurement of mononuclear cells was examined as indicators of magnesium status in a small number of studies involving human diseases associated with magnesium deficiency. Results from notable studies have been reviewed (Arnaud, 2008). Among the findings were the following: a significant correlation between magnesium concentrations in lymphocytes and muscle in patients with type 1 diabetes; lower lymphocyte ionized magnesium concentrations in migraine patients than in controls; lower lymphocyte total magnesium content in hypertensive patients than in controls; and a decrease in lymphocyte magnesium content in patients with congestive heart failure experiencing arrhythmias because of digitalis toxicity. Magnesium concentrations in platelets were also examined in patients with diabetes, obesity, and hypertension but were found not to be a more sensitive nor a more specific marker of magnesium status than other cellular magnesium measurements (Arnaud, 2008). There is no reference range for cellular mononuclear magnesium. Only a few studies have indicated the normal concentration of magnesium in mononuclear cells. For example, a mean of 2.91±0.6fmol Mg/cell was obtained from 20 apparently healthy adults (Elin & Hosseini, 1985), whereas expressed as μmol/mg protein, mean cellular mononuclear magnesium concentrations ranged from 0.052 to 0.073μmol/mg protein (Elin & Johnson, 1982; Hosseini et al., 1983; Sjögren et al., 1986). Although cellular mononuclear magnesium appears to be a better indicator of magnesium status or stores than serum total or ionized magnesium, several drawbacks preclude the use of cellular mononuclear magnesium as a routine clinical measure. For example, large blood samples are required for fractionating cellular components. Moreover, the fractionation method used to obtain the specific cell types is difficult, time consuming, and prone to errors. Analysis of magnesium concentrations in the specific cell types is usually performed by atomic absorption spectrometry.23c.8.5 Urinary magnesium
Urinary magnesium excretion is an excellent indicator of magnesium intake and is usually measured by atomic absorption spectrometry. Urinary magnesium excretion data obtained from 27 metabolic unit studies have shown that when intakes were 200mg/day or lower, urinary magnesium excretion generally ranged from 40‑80mg/day (1.65‑3.29mmol/day). When dietary intakes were greater than 250mg/day, urinary magnesium excretion ranged from 80‑160mg/day (3.29‑6.58mmol/day) (Nielsen & Johnson, 2017). Four depletion-repletion experiments involving metabolic unit studies with postmenopausal women found that urinary magnesium excretion was between 40‑80mg/day (1.65‑2.88mmol/day) with dietary magnesium intakes less than the EAR suggested for the United States and Canada of 175mg/day (Nielsen & Johnson, 2017). With magnesium intakes above 300mg/day, urinary magnesium excretion ranged from 100‑140mg/day (4.11‑5.76mmol/day) The four depletion-repletion experiments also found that urinary magnesium excretion responded quickly to changes in dietary magnesium. For example, in one study, women who consumed a mean magnesium intake of 390mg/day had a mean daily urinary excretion of 120‑130mg/day) (4.93‑5.35mmol/day). When the women were switched to a diet supplying a mean magnesium intake of 120mg/day, mean urinary magnesium excretion decreased to 63mg (2.59mmol) per day within 6 days. A switch from a magnesium deficient intake to an adequate intake resulted in a similar change in urinary magnesium excretion within 6 days. These findings indicate that a single 24‑hour urine sample or short-term determination of 24‑hour urinary magnesium excretion alone is not useful as an indicator of current magnesium status at the individual level because urinary magnesium excretion could be low while an individual still has an adequate magnesium status and vice versa. However, at the population level, urinary magnesium excretion could be an indicator of the amount of inadequate magnesium status in a population. This suggestion is supported by the findings that low urinary magnesium excretion has been associated with the risk of cardiovascular disease (Joosten et al., 2013a; Yamori et al., 2015) and hypertension (Joosten et al., 2013b). Urinary magnesium excretion can also be useful in magnesium status assessment when combined with other measures of magnesium status. As indicated above, using a urinary magnesium excretion of < 80mg/day combined with a dietary magnesium intake < 250mg/day could increase the likelihood of identifying individuals who are magnesium-deficient even though serum magnesium concentrations are within the low healthy reference range. This suggestion is supported by a recent finding that young women with a mean urinary magnesium excretion of 58.2mg/day, a dietary magnesium intake of 145mg/day, and a normal serum magnesium responded to magnesium supplementation by increasing urinary magnesium excretion (Okamoto et al., 2023).23c.8.6 Magnesium load test
The magnesium load test is considered by many to be the best method for determining total body magnesium status or general intracellular magnesium content. The basis for the magnesium load test is that the kidney regulates the body stores of magnesium by excreting excess magnesium when stores are adequate and retaining magnesium when stores are deficient. This test determines the percentage of magnesium retained over a given period time after parenteral administration of a magnesium load (Elin, 2010; Rude & Shils, 2006). Retention of a greater percentage of magnesium than that retained by individuals with adequate magnesium status indicates some depletion of body magnesium. The test depends upon normal renal function; it cannot be used on individuals with renal diseases or who are taking drugs that affect renal function. The magnesium load test has been used to identify magnesium deficiency in elderly individuals, and in conditions of chronic alcoholism, ischemic heart disease, hypertension, and Crohn's disease (Waters et al., 2008) where hypomagnesemia was not always found. To perform this test properly, a basal urinary magnesium excretion is determined. Three consecutive 24‑hour urine samples should be collected to yield the most accurate basal magnesium excretion and counteract the effects of both diurnal and random day-to-day variations in magnesium excretion. Nevertheless, measurement of a single 24‑hour basal urinary magnesium excretion collected just before the magnesium load is most often used because it has been found to give acceptable results. After the determination of the basal urinary excretion, magnesium is administered parentally instead of orally to eliminate variability that would occur with intestinal absorption. A slow infusion, usually done over a period of 4 to 8 hours, is needed because plasma magnesium concentration determines the renal reabsorption threshold. A sudden increase in plasma magnesium above the normal level would increase urinary excretion and thus reduce magnesium retention, which could result in an erroneous assessment of status. Following the load, urinary excretion of magnesium is measured over the subsequent 24 to 48‑hour period. Although a 48‑hour urine collection gives a slightly more accurate measure of magnesium retention, 24‑hour urine collections have been found to correlate well with 48‑hour urine collections (Gullestad et al., 1994) so are often used. Urine specimens should be collected with an acidifying agent to prevent precipitation of magnesium compounds at high pH. The net retention of magnesium is calculated by comparing basal magnesium excretion with net magnesium excretion after the load. A standard protocol for administering the magnesium load has not been established. Examples of protocols reported include a 30mmol (720mg) of magnesium sulfate in 1000ml of isotonic saline for 8 hours at a rate of 125ml/hour (Gullestad et al., 1994); an intravenous infusion of 30 mmol (720mg) of magnesium in 500ml 5% dextrose over a period of 8‑12 hours (Ismail et al., 2012) and an intravenous infusion of 0.2mmol/kg body weight (340mg for a 70kg individual) of magnesium sulfate in 250ml of 5% dextrose over 4 hours (Ozono et al., 1995). The percentage magnesium retention is calculated as:
[(Amount of Mg infused)
− (post-infusion urinary Mg)
− (baseline urinary Mg)] x 100%
divided by
(Amount of Mg infused)
(DiNicolantonio et al., 2018).
The infusion protocols outlined above gave similar results in terms
of suggesting the magnesium retention indicating magnesium adequacy and deficiency.
A retention reference range providing the values indicating deficiency and adequacy has not
been officially established. However, it has been suggested that
a retention of < 20% indicates
adequate magnesium status; retention between > 20% to > 50% indicates
borderline deficient
magnesium status; and retention > 50% indicates magnesium deficiency
(Costello & Nielsen, 2017).
The borderline deficiency values, which would indicate CLMD, are apparently
appropriate because individuals with values within this range reportedly had chronic diseases
associated with a low magnesium status but did not exhibit hypomagnesemia (i.e., low serum
magnesium concentrations).
The magnesium load test is invasive, time-consuming, and cumbersome; it requires close
supervision for at least 24 hours after magnesium infusion to ensure oversight of both the total
amount of magnesium infused and the urine collection. These drawbacks prevent the
magnesium load test being a routine clinical measure of magnesium status. It has been used
most often as a research tool to determine whether individuals with chronic disease are
magnesium deficient.
− (post-infusion urinary Mg)
− (baseline urinary Mg)] x 100%
divided by
(Amount of Mg infused)
23c.8.7 Muscle magnesium
Because muscle contains about 30% of the total body magnesium, muscle magnesium has been evaluated as a possible indicator of magnesium status. Very few studies have found a correlation between concentrations of magnesium in muscle and serum or erythrocytes (Elin, 1991). However, some significant correlations with mononuclear blood cells have been found in some disease states such as type 1 diabetes (Sjögren et al., 1986) and mild hypertension (Dyckner & Wester, 1985), which suggests that muscle magnesium may be a reliable indicator of intracellular magnesium status. This suggestion is supported by a metabolic unit study with postmenopausal women in which magnesium depletion by dietary means significantly decreased muscle magnesium, whereas repletion restored muscle magnesium levels (Lukaski & Nielsen, 2002). In the magnesium depletion-repletion metabolic study outlined above, 40mg of muscle tissue was obtained by needle biopsy. After adipose and connective tissue were dissected from each specimen, the specimen was rinsed with distilled-deionized water and dried and digested with nitric acid. Magnesium was determined by inductively coupled plasma emission spectrometry. Muscle magnesium concentrations were 53.4, 48.1, and 51.6mmol/kg dry weight during the control, depletion, and repletion periods, respectively. However, the procedure to obtain muscle samples is extremely invasive and requires skill to obtain a sample with minimal fat and without causing a hematoma. Proton nuclear magnetic resonance spectroscopy has also been used to measure ionized magnesium in skeletal muscle (Reyngoudt et al., 2019). However, this method of measurement is very expensive and requires the use of complex instruments and sophisticated software, which precludes its use in specialized laboratories only. Because other methods, such as the magnesium load test, and erythrocyte membrane and mononuclear cell magnesium determinations give status information of similar validity, using muscle magnesium to assess status in either the clinical or research setting is not useful.23c.8.8 Buccal call magnesium
intracellular magnesium in sublingual epithelial cells was found to correlate with magnesium in atrial cell biopsies from open heart surgery patients (Haigney et al., 1995). Hence, the measurement of magnesium in sublingual epithelial cells has been suggested as a method that can assess CLMD (Silver, 2004). The cells are collected by gentle scratching of sublingual tissue and then fixed on a carbon slide with a cytology fixative. The magnesium in the fixed cells is determined by using energy-dispersive X-ray analysis. However, the test of magnesium in sublingual epithelial cells as a measure of magnesium status needs to be validated by using another test such as the magnesium-load test. Furthermore, the method is expensive and requires skill in collecting suitable sublingual epithelial cell samples for analysis, which has limited its use mainly to research laboratories.23c.8.9 Magnesium status assessment methods of limited use
Magnesium balance (intake minus loss) is the principal measure used to determine dietary magnesium requirements. Most balance studies have been performed in clinical research centers. The method requires an accurate determination of magnesium from dietary intake and from the complete collection of urine and stool over at least 12 days. It also should include the collection and measurement of sweat, dermal, and menstrual losses of magnesium, although generally these losses have not been measured in reported balance studies. A negative balance (i.e., magnesium losses exceed magnesium intake) indicates a magnesium deficiency, and a positive balance (i.e., magnesium intake exceeds magnesium losses) indicates adequacy. Most reported balance studies prior to 1990 did not have well-controlled dietary intakes of magnesium, used short periods of data collection, and/or used less accurate methods to measure magnesium. A poorly controlled magnesium balance study was used to set the DRIs for magnesium in the United States and Canada (IOM, 1997). The most valid balance studies have involved individuals in a controlled metabolic unit environment who have been provided with a controlled and consistent diet (Hunt & Johnson, 2006; Nielsen, 2016). Such studies have indicated an EAR of 175mg/day for healthy 70kg individuals. Fractional excretion of magnesium (FEMg) distinguishes between gastrointestinal and renal magnesium loss. This method has received some brief mentions as a measure of magnesium status (Ayuk & Gittoes, 2014; Costello & Nielsen, 2017). The following formula is used to determine this measure (Ayuk & Gittoes, 2014): FEMg= [(uMg × sCr)/(sMg × uCr × 0.7)] × 100%where uMg = urinary Mg concentration; sMg = serum Mg concentration; sCr= serum creatinine; uCr = urine creatinine Fractional excretion of magnesium (FEMg) greater than 2% is considered an indication of renal wasting which implicates extra-renal magnesium losses, decreased intake, or decreased absorption of magnesium. As this method of assessment requires the determination of three variables, it provides no advantage to assess magnesium status over the use of serum magnesium, ionized magnesium, or the magnesium load test and hence has received limited application as a measure of magnesium status. Magnesium depletion score (MDS) is an aggregate of several risk factors affecting the absorption and excretion of magnesium (Fan et al., 2021; Wang et al., 2022). The MDS is used to identify individuals with abnormal magnesium absorption and/or excretion that may result in a deficient magnesium status. The MDS is calculated by aggregating the factors:
- Current use of diuretics counted as 1 point;
- Current use of proton pump inhibitor counted as 1 point;
- Heavy drinker (defined as > 1 drink/day for women and > 2 drinks/day for men) counted as 1 point;
- Mildly decreased kidney function defined as estimated glomerular filtration rate (eGFR) 60mL/(min × 1.73m2) < eGFR 90ml/min × 1.73m2 counted as 1 point;
- Chronic kidney disease defined as eGFR < 60ml/min × 1.73m2 counted as 2 points.